The Upright Thinkers by Leonard Mlodinow—former professor of Physics at Caltech and author of several other best selling books on science—tells his version of “the human journey from living in trees to understanding the cosmos.” The story is epochal. We begin by noting several of Mlodinow’s general themes.
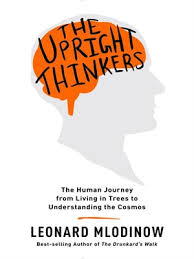
Mlodinow asserts that our odyssey of discovery has been driven by our inborn and virtually insatiable curiosity; our propensity to ask “why?” “Human children all around the world ask their first questions at an early age, while they are still babbling and don’t yet speak grammatical language…Chimpanzees and bonobos, on the other hand, can learn to use rudimentary signing to communicate with their trainers, and even answer questions, but they never ask them. They are physically powerful, but they are not thinkers.”
We also are reminded that our astonishing progress was facilitated by a unique characteristic of our species—we add to knowledge already in existence. Recall the famous quote of Isaac Newton, “”If I have seen further than others, it is by standing upon the shoulders of giants.”
Related to the above, there is archaeological evidence that schools existed as early as 2,500 B.C. “…the idea that society should create a profession devoted to passing on knowledge, and that students should spend years acquiring it, was something entirely new—an epiphany for our species.”
Another of Mlodinow’s themes is that the major players in our quest to understand the cosmos (e.g., Newton, Mendeleev, Darwin, Einstein, Bohr, and Heisenberg) were not merely brilliant. They were also stubbornly persistent individuals. Contrary to popular belief, the groundbreaking discoveries they made did not result from sudden “aha moments” but, instead, came about only after years of dogged hard work. There was no apocryphal apple that serendipitously landed on Newton’s head.
Mlodinow also reminds us that scientists, as well as laypeople, do not easily to let go of the conventional beliefs of their day, even when faced with strong evidence to the contrary. Consequently, new scientific paradigms, even when supported by incontrovertible evidence, are often initially rejected by the establishment. And since the revolutionary breakthroughs that Mlodinow highlights in his tale indeed challenged the established wisdom of their times, the scientists who put them forward had to posses more than a little courage and even audacity to complement their doggedness. [Howard Temin provides a recent example of a virologist who persevered in the face of scorn and ridicule from his colleagues. See Howard Temin: “In From the Cold,” posted on the blog December 16, 2013.]
Mlidinow allocates several pages to the origin and acceptance of the concept of universally applicable natural laws. The idea of natural laws already existed in ancient Greece. However, Greek natural laws were each specific to a particular situation. Our modern concept of universally applicable natural law came into being only in the early seventeenth century, as prompted by the discoveries of Kepler, Newton, and their contemporaries (see below).
Why might it have taken mankind so long to recognize that nature acts according to certain regularities? The answer may lie in the fact that when primitive humans were faced with seemingly inexplicable droughts, floods, plagues, earthquakes, and so forth, it was difficult for them to view the world as anything but chaotic. Remarkably, even today there still are those who reject the idea of a universe ruled by natural laws. [Consider this. Mlodinow relates that Einstein was astonished by the fact that nature has order. Einstein wrote: “one should expect a chaotic world, which cannot be grasped by the mind in any way… the most incomprehensible thing about the universe is that it is comprehensible.”]
Returning to the theme of our difficulty transitioning from judging the truth of a statement according to how well it fits convention or religious belief, to how well it is supported by empirical evidence, note that for nearly two millennia scientific progress was impeded by the conventions of ancient Greek science. Contrary to popular belief, the Greeks did not invent the scientific method (2). Nor were their theories developed with the intent of experimental verification. Aristotle’s science held that nature did what pure logic suggested it should do. For example, Aristotle said that heavier objects fall faster than lighter objects, because it is their purpose to do so. Neither Aristotle nor his contemporaries actually looked to see if heavier objects indeed fall faster than lighter ones. In fact, the first individual known to have actually tested this premise was Galileo (1564-1642). Only afterwards did observation and experimentation become the basis for western science.
Despite the fact that Aristotle’s non-quantitative search for purpose impeded scientific progress for nearly two thousand years, Mlodinow credits him with applying reason in the pursuit of understanding and, also, for the idea that nature acts according to certain regularities. Mlodinow also notes that Aristotle was hamstrung by the technology of his day. In particular, since Aristotle did not have a stopwatch, concepts such as velocity and acceleration—keystones of Newtonian physics—may have been beyond his reach. But, “More important was the fact that Aristotle was, like everyone else, simply not interested in quantitative description.”
Notwithstanding the above, why did the leap from Aristotelian physics to the breakthroughs of Galileo and Newton take 2,000 years? According to Mlodinow, the conquest of Greece by Rome was a key reason. The Romans were superb engineers, but they had little interest in the pursuit of knowledge for its own sake. Thus, the Roman conquest of the Greeks resulted in the fading out of the Greek scientific heritage from Western culture.
At the end of the eleventh century, a Benedictine monk, Constantinus Africanus, translated ancient Greek medical texts (preserved for centuries by Muslim court scholars) from Arabic to Latin. This was a first step in the revival of the Greek scientific heritage in Europe. Moreover, it was a step towards the eventual resumption of Western science (see Aside 1). Additionally, the development of European universities, such as Oxford (by 1250), facilitated scientific progress in the West, since—then as now—universities are a place that brings people together, where they might interact with and stimulate one another. [Most scientific progress still emanates from universities.] The emergence of moveable-type printing at around 1450 was another key development, since it enabled the widespread dissemination of new ideas.
[Aside 1: Intellectual progress in the Islamic world and in China often surpassed that in Europe during the Middle Ages. Why then did modern science not emerge in the Islamic world, or in China, instead of in Europe? For an answer, I turn briefly to Why the West Rules—For Now by Ian Morris (3). Morris tells us that the resurgence of science in Western Europe was largely driven by Europe’s “new frontier” across the oceans, and the need it created for the precise measurement of time and space. Moreover, “…by the point that two-handed clocks had become the norm Europeans would have to have been positively obtuse not to wonder whether nature itself was not a mechanism.” Additionally, the industrial revolution in the West, which was largely driven by the Atlantic economy, stimulated further mechanical invention and scientific development.]
Galileo was one of the most influential scientists who ever lived and a key figure in the resurgence of European science. One reason for his fame was his experiment at the Leaning Tower of Pisa, which showed that light objects fall as rapidly as heavy ones. Yet while he was disenchanted by the lack of experimentation in Aristotelian physics, he did not invent experimental physics per se. However, his quantitative approach to experimentation was indeed revolutionary. Moreover, “when he got a result that surprised him or went against conventional thinking, he didn’t reject it—he questioned convention and his own thinking.”
One of Galileo’s most insightful and important contentions was that objects in uniform motion tend to remain in that state of motion. That is, an object does not require the continuous application of a force to remain in motion; an assertion that was contrary to the prevailing Aristotelian view that objects require a continuing reason for their motion. If Galileo’s assertion sounds oddly familiar, it is because Newton later adapted it as his first law of motion. [“A few pages after stating the law, Newton adds that it was Galileo who discovered it—a rare instance of Newton giving credit to someone else.”]
Galileo is also famous for his conflict with the Catholic Church over his claim that the Earth is not at the center of the universe. But the idea of a heliocentric (i.e., sun-centered) universe did not originate with Galileo. In fact, it existed as early as the third century B.C. in Greece, and a “modern” European version can be attributed to Copernicus (1473-1543). But Galileo was the first to offer irrefutable proof that the Earth is not at the center of the universes. What’s more, Galileo made his case compelling only after he perfected the telescope, which enabled him to achieve unprecedented levels of power..
Isaac Newton was born in 1642; the year that Galileo died. He is commonly said to have invented the calculus, and to have discovered his laws of motion and universal gravitation, all in the single year, 1666. The last discovery supposedly followed his serendipitous encounter with a falling apple. The reality was a bit different. First, the calculus was concurrently and independently invented by Leibnitz. Second, Newton labored continuously for a period of three years—1664 through 1666—in order to discover his laws of motion. Yet even after accomplishing that feat, Newton was still not a Newtonian. “He still thought of uniform motion as arising from something internal to the body, and by the term ‘gravity’ he meant some inherent property arising from the material an object is made of , rather than an external force exerted by the earth.”
After discovering his laws of universal motion, Newton spent additional years acquiring information, largely compiled by others, regarding planetary orbits. Kepler’s finding—that planetary orbits are ellipses, with the sun at one of the foci—would be especially significant, as follows.
Edmond Halley (the astronomer who computed the orbit of the comet bearing his name), Robert Hooke (the discoverer of cells and perhaps the greatest experimentalist of the seventeenth century), and Christopher Wren (best known as an architect, but also an accomplished astronomer) gleaned from Kepler’s data a premise of singular importance; that the pulling force of the sun diminishes in proportion to the square of the planet’s distance from it. Yet Halley, Hooke, and Wren were unable to prove their conjecture.
Halley, happening to be in Cambridge, took the opportunity to visit Newton. During his visit with Newton, Halley informed his host of the conjecture concerning the pulling force of the sun. Imagine Halley’s surprise when Newton claimed that he had already discovered the premise and, indeed, had already proven it. Newton then set about looking for his proof. But when he found it, he discovered that it was in error. He then set about reworking his proof and, in so doing, he confirmed that Kepler’s planetary orbits indeed are explained by an inverse square law of attraction.
Newton’s proof of the inverse square law of gravitational attraction was followed by more hard work that resulted in “what is perhaps the most significant intellectual discovery that had ever been made”—Newton’s universal theory of motion, which showed that free fall and orbital motion are instances of the same laws of force and motion. Newton set down his laws of motion, and his law of universal gravitation, in his famous Principia; three books published in 1687, which are regarded as the foundation of classical mechanics and, thus, as one of the most important works in the history of science. Halley personally paid the cost of publishing the Principia.
Newton died in 1726. “Newton’s life and Galileo’s had together spanned more than 160 years, and together they witnessed—and in most respects accounted for—most of what is called the scientific revolution.”
A revolution in chemistry was occurring concurrent with the ones in physics and astronomy. The key breakthrough in chemistry would be the discovery that some substances—the elements—are fundamental, and that all else is made from them. Actually, the concept of elements dates back to Aristotle and the ancient Greeks. However, Aristotle’s chemistry had but four “elements”—earth, air, fire, and water.
The experimental results of Robert Boyle (1627-1691) were the first to free chemistry from Aristotelian convention. Mlodinow relates how in 1642, the 15-year-old Boyle was taken with science after reading Galileo’s book on the Copernican system. Afterwards, the adult Boyle (incidentally assisted by Robert Hooke) investigated respiration and combustion. His findings led him to conclude that air is not an element but, instead, is made up of different substances. Moreover, Boyle’s quantitative approach set in place a new convention in chemistry of careful experimental analysis.
Boyle’s experiments were followed by those of Joseph Priestly (1733-1804) and of Antoine Lavoisier (1743-1794). The relationship between these two men makes for an interesting tale, one that is too long to review here. But, together, their researches showed that respiration and combustion removed something from the air. Priestly may have discovered it first, but Lavoisier gave it its name, oxygen. Lavoisier went on to reveal one of chemistry’s major principles—the conservation of mass. That is, the total mass of the products produced in a chemical reaction must be the same as the combined mass of the initial reactants.
Lavoisier had been a tax collector in Paris in the days preceding the French Revolution. His day job provided financial support for his experiments, but it did not endear him to the revolutionaries who overthrew the French monarchy. He was arrested in 1793 during the Reign of Terror and sentenced to death. The presiding judge is said to have told him, “The Republic has no need of scientists.” “By the time of his execution, Lavoisier had identified thirty-three known substances as elements. He was correct about all but ten.”
The next important advancements would involve the nature of atoms. Although Lavoisier identified the elements as the reactants in chemical processes, he chose not to think in terms of atoms. His reason was simply that he did not know of any way by which something as tiny as an atom could be observed or experimented on. John Dalton (1766-1844) took the first step towards solving that problem when he discovered how to determine atomic weights by applying Lavoisier’s law of conservation of mass. [Mlodinow details Dalton’s approach.]
Dmitry Mendeleev (1834-1907) made a crucial breakthrough that would lead to an understanding of the relationship between the weight of an atom and its chemical properties. [The relationship of course involves atomic numbers, rather than atomic weights. Please be patient.] How that development came to pass was one of my favorite anecdotes in Mlodinow’s book. It was as follows. In 1866, while Mendeleev was a chemistry professor in St. Petersburg, he could not find a first-rate, up-to-date chemistry book, written in Russian, that he might use in his teaching. So, he decided to write his own. Mendeleev’s book was a labor of love that he worked on for years. Early on, he wrestled with the question of how to organize the book. His solution was to arrange the elements and their compounds into groups, as defined by their properties. Then, after much arranging and rearranging of the elements, he discovered a pattern that we know today as the “periodic table;” a discovery of singular importance that Mlodinow calls “chemistry’s version of Newton’s laws.”
Much important work remained to be done. Because there were as yet undiscovered elements, there were gaps in the Mendeleev’s table. Moreover, some of the assigned atomic weights were wrong and, crucially important, the chemical properties of an element are determined by the as yet undiscovered atomic numbers, rather than by atomic weights. Notwithstanding that, to Mendeleev’s credit, he accurately predicted the existence and chemical properties of the elements which corresponded to the gaps in his table.
The twentieth-century revolution in physics led to profound insights into the nature of space and time, and to a deeper “understanding” of the atom. Mlodinow tells that story only after he reviews several key breakthroughs in biology. Keeping with his format, he notes the early influence of Aristotelian convention on biology. Two Aristotelian beliefs were especially important. One was the belief that a divine intelligence designed all living beings. The other was the belief in spontaneous generation.
Readers of the blog are likely familiar with Louis Pasteur’s late nineteenth-century experiments, which laid spontaneous generation to rest once and for all (4). Many readers may also know that Francesco Redi experimentally challenged spontaneous generation more than two centuries earlier. In 1668, Redi’s microscopic observations of minute organisms showed that they are much more complex than had been imagined. In particular, these organisms had reproductive organs, which called into question one of Aristotle’s arguments for spontaneous generation; that lower organisms are too simple to reproduce.
The notion, that a divine intelligence was necessary to design all living beings, was a foundation stone of nineteenth-century thinking. Under that circumstance, Charles Darwin (1809-1882) gave the world a scientific theory—evolution by natural selection— that explained how species came to have their particular characteristics. Darwin’s theory directly challenged the biblical account of creation, which still has a very significant number of adherents. Thus, it is all the more remarkable that his grave occupies hallowed ground under Westminster Abbey.
Legend has it that Darwin had an “aha moment” while observing the beaks of finches on different islands of the Galapagos. The reality is that Darwin only gradually came to believe that species are not unchanging forms designed by God but, instead, evolved over time. And Darwin devoted many more years to discovering the mechanism behind evolution—natural selection. Moreover, knowing that his findings would expose him to ridicule and attack, he spent more years amassing incontrovertible evidence in support of his theory.
Darwin did not know of genes and mutations. Consequently, he was unaware of how genetic variability might provide the raw material for adaptation. His contemporary, Gregor Mendel (1822-1884), made the breakthrough in genetics. Mendel, carried out his experiments while a monk at a monastery in what is now the Czech Republic, perhaps explaining why Darwin never knew of his work.
One way or another, serendipity is usually a factor in all great scientific discoveries. Apropos the theory of evolution, Darwin likely would have remained an unknown English churchman were it not for the unlikely and unexpected letter inviting him, at twenty-two years of age, to sail around the world as a naturalist on the Beagle. The position on the Beagle was beforehand offered to a number of others, none of whom were willing to spend two years at sea.
Mlodinow devotes the last 100 or so pages of his book to the twentieth-century revolution in physics. Considering the stunning discoveries that were about to unfold, it is more than a bit ironic that the prevailing belief at the start of the century was that everything in physics was already known and that nothing else remained to be done.
Key individuals who fill Mlodinow’s final pages include Max Planck (see Aside 2), Albert Einstein (see Aside 3), Ernest Rutherford (see Aside 4), Niels Bohr (see Aside 5), and Werner Heisenberg and Erwin Schrodinger (see Aside 6).
[Aside 2: Max Planck was a German physicist who deduced the relationship between the frequency of radiation and its energy. Moreover, he proposed that electromagnetic energy could only take on discrete values, or quanta. In so doing, he originated quantum theory.
Mlodinow tells the following quip: “Since my name is so hard to spell and pronounce, when I make a restaurant reservation, I often do it under the name Max Planck. It’s very rare that the name is recognized, but one time when it was, I was asked if I was related to the “guy who invented quantum theory.” I said, “I am that man.” The maitre d’, in his early twenties didn’t believe me. He said I was too young. “Quantum theory was invented around 1960,” he said. “It was during World War II, as part of the Manhattan Project.”]
[Aside 3: In the single year 1905, while Einstein was employed as a third-class clerk at the Swiss patent office in Zurich, he published three singularly important, ground-breaking papers. The first paper explained Brownian motion, the second the photoelectric effect (in which light impinging on a metal causes it to emit electrons), and the third, his discovery of special relativity. Although each of the three papers dealt with a different topic, astonishingly, each was important enough to merit a Nobel-Prize. And, since Einstein is best known for his theories of relativity—which revolutionized our concepts of space and time—it may surprise some that his Nobel Prize was actually awarded for his explanation of the photoelectric effect. Einstein’s key insight was to treat light as a quantum particle, rather than as a wave. Consequently; his analysis of the photoelectric effect would significantly advance further developments in quantum theory; which Einstein eventually came to abhor (see below). His explanation of Brownian motion provided the most convincing evidence of the day for the existence of atoms. After years more of work, Einstein put forth his theory of general relativity, which incorporated gravity into relativity theory.]
[Aside 4: Rutherford discovered of the structure of the atom, with its positive charge concentrated in the nucleus. Interestingly, this key advancement began fortuitously, as a “small research project,” for a young undergraduate student named Ernest Marsden; for him “to get his feet wet.”]
[Aside 5: Bohr applied quantum mechanics to the whole atom, asking what it might mean if the atom, like light quanta, could have only certain energies. His premise, that electrons occupy only certain allowable orbits, provided an explanation for why electrons don’t spiral into the atom’s nucleus. And his proposal that the atom’s outer orbits determine its chemical properties, gave new insights into why Mendeleev’s heretofore mysterious periodic table worked.]
[Aside 6: Heisenberg and Schrodinger proposed dissimilar quantum theories, each of which left behind Newton’s conventional description of reality. In Newton’s physics, position and velocity are independently measured quantities, one event causes another, and the world exists independently of our observation of it. In quantum theory, reality is based on probabilities and uncertainties, and reality does not exist independently of our observations. At a scientific conference, when Einstein famously attacked the indeterminacy and probabilistic nature of quantum physics, saying “God does not play dice with the universe,” Bohr famously replied, “Einstein, stop telling God what to do (5).” Yet even Schrodinger eventually turned against quantum theory, offering up his famous fable of the cat that was neither alive nor dead, to point up the seeming absurdity of the theory. Still, quantum theory remains as the most predictive of all scientific theories.]
The Nazis came to power in Germany in 1933. They hated the new physics for being surreal and abstract but, more importantly, because they considered it to be the work of scientists of Jewish heritage (e.g., Einstein, Born, Bohr, Pauli). [Incidentally, the new physics was also condemned in the Soviet Union in the early 1920s; in that instance for not conforming to the principles of Marxist-Leninist ideology. The Soviets similarly banned Mendelian genetics for the same reason.]
Einstein was still living in Germany when the Nazis took control. But, fortunately, he was visiting Cal Tech on the very day in January 1933 when Hitler became Chancellor. He never returned to Germany. His property in Germany was confiscated, his notes on relativity were burned, and a five-thousand-dollar bounty was put on his head.
Heisenberg tried to accommodate himself to the Nazis, but he was nevertheless harassed by them for having worked on “Jewish physics” with Jewish physicists, and for trying to have Max Born (incidentally Max Delbruck’s mentor) exempted from the Nazi non-Aryan work prohibition. Under pressure, Heisenberg disavowed the Jewish physicists and “their” science.
Max Born, with the help of Pauli’s refugee organization, escaped to Cambridge. Schrödinger, an Austrian who had been living in Berlin when Hitler came to power, was an outspoken anti-Nazi. He left Berlin to take a position at Oxford. Bohr’s real-life exploits during the Nazi era are more fascinating than fiction, and are covered in some detail in reference (5).
In 1933, Max Planck met face-to-face with Hitler to dissuade him from carrying out the anti-Jewish policies that were causing top Jewish scientists to leave Germany. The meeting came to nothing, and Planck quietly went on with his work. However, Planck’s youngest son was a member of the failed plot to assassinate Hitler on July 20, 1944. He was arrested by the Gestapo and was executed along with the other conspirators. Earlier, in 1907, when women were nearly entirely barred from receiving advanced education, Planck invited Lisa Meitner (only the second woman to receive a physics doctorate from the University of Vienna) to carry out postdoctoral studies under his guidance (5). Mlodinow includes Meitner among the short list of physicists who he believes deserved, but did not receive the Nobel Prize. See Aside 6. [Reference 6 notes the wartime experiences of Andre Lwoff, Francois Jacob, Jacques Monod, and Elie Wollman. Reference 7 describes a wartime experience of Renato Dulbecco.]
[Aside 7: By 1938, Lisa Meitner’s situation in Nazi Germany had become desperate. So she fled to Holland, aided by Dutch physicists who persuaded their government to admit her on her Austrian passport, which was no longer valid after the Anschluss (5). She next moved to Sweden, where Niels Bohr found for her a laboratory where she could continue her work. While in Sweden, she received a correspondence from her former collaborator in Germany, chemist Otto Hahn, from which she and her nephew Otto Frisch calculated that Hahn had unknowingly witnessed nuclear fission (5). Meitner passed that information on to Niels Bohr, which Bohr took to America and made public at a January 1939 conference at George Washington University. Leading proponents of the new physics immediately realized that a nuclear bomb was now possible. The famous Einstein letter of August 1939, which warned Franklin Roosevelt of that possibility, led to the American Manhattan Project to develop an atomic bomb. German scientists, led by Heisenberg, likewise began work on nuclear energy. In September 1939, Germany invaded Poland and World War II was underway. By September, 1941, Britain was fighting alone against Germany in the west, and the Soviets were reeling under the German onslaught in the East. Those were the circumstances under which Heisenberg attended a German-sponsored conference in Nazi-occupied Copenhagen. Bohr boycotted the conference in protest against the Nazis. However, Heisenberg sought out his former mentor in Copenhagen for a private meeting. While it is known that Bohr and Heisenberg discussed the nuclear weapon issue, the two men were never able to agree on exactly what was said, or even where their conversation actually took place, and to this day their meeting remains shrouded in mystery. Perhaps because Bohr was potentially a huge scientific asset to the Germans, or perhaps because his life was in danger because of his Jewish heritage, the British in 1943 carried out a harrowing rescue mission to smuggle him out of occupied Denmark. Details of the rescue are recounted in reference 5. Bohr then came to America, where he worked on the Manhattan Project and he later became an outspoken arms control advocate. Although it is not entirely clear, there are reasons to believe that Heisenberg may have surreptitiously impeded the German nuclear effort. Bohr and Heisenberg reestablished their relationship after the war, but their shaky friendship was sustained only by their mutual understanding not to revisit their 1941 meeting.]
Mlodinow asserts that “quantum theory was created by a concentration of brain power in Central Europe that surpassed or at least rivaled that of any of the intellectual constellations we’ve encountered in our journey through the ages.” Moreover, “It was a magical time in Europe, with burst after burst of imagination lighting the sky, until the outline of a new realm of nature began to appear.”
A photo in The Upright Thinkers, taken at the famed 1927 Fifth Solvay International Conference on Electrons and Photons, shows Erwin Schrodinger, Wolfgang Pauli, Werner Heisenberg, Paul Dirac, Max Born, Niels Bohr, and Albert Einstein among others; thus bearing out Mlodinow’s contention that it was a very special time in the history of science.
The “golden age of molecular biology” of the 1950s and 1960s is not discussed in The Upright Thinkers. Thus, I feel an urge to proclaim before closing that it too was a very special time in the history of science, when fundamental discoveries were made in biology that were comparable in significance to those made earlier in the century in physics . As a graduate student in the 1960’s I was privileged to experience the thrill of attending, and speaking at the summer conferences at Cold Spring Harbor, which were simultaneously graced by James Watson, Francis Crick, Max Delbruck, Sidney Brenner, Francois Jacob, Jacques Monod, Seymour Benzer, and Norton Zinder (all of whom are discussed elsewhere on the blog), as well as by the likes of Erwin Chargaff, Matthew Meselson, Frank Stahl, Sol Spiegelman, John Beckwith, and so forth.
This piece at last concludes with an item from The Upright Thinkers, which I found to be especially intriguing. Recent DNA analysis shows that around 140,000 years ago, a catastrophic event—possibly related to climate change—caused the entire human population to plummet to a mere several hundred individuals. We truly were an endangered species. “Isaac Newton, Albert Einstein, and everyone else you’ve ever heard of, and the billions of us who live in the world today, are all descendants of those mere hundreds who survived.”
References:
(1) Leonard Mlodinow, The Upright Thinkers, Pantheon Books, 2015.
(2) Thucydides and the Plague of Athens, posted on the blog September 30, 2014.
(3) Ian Morris, Why the West Rules—For Now, Farrar, Straus and Giroux, 2010.
(4) Louis Pasteur: One Step Away from Discovering Viruses, posted on the blog January 7, 2015.
(5) Max Delbruck, Lisa Meitner, Niels Bohr, and the Nazis, posted on the blog November12.
(6) Genealogies and a Selective History of Lysogeny: Featuring Friedrich Loeffler, Emile Roux, Andre Lwoff, Elie Wollman, and Francois Jacob, posted on the blog January 28, 2015.
(7) Renato Dulbecco and the Beginnings of Quantitative Animal Virology, posted on the blog December 3, 2013.